Category: Profilometry | Texture and Grain
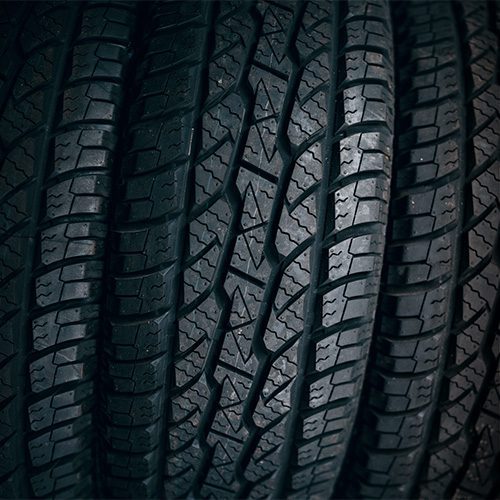
Rubber Tread Contour Measurement
Learn More
RUBBER TREAD CONTOUR MEASUREMENT
USING 3D OPTICAL PROFILER
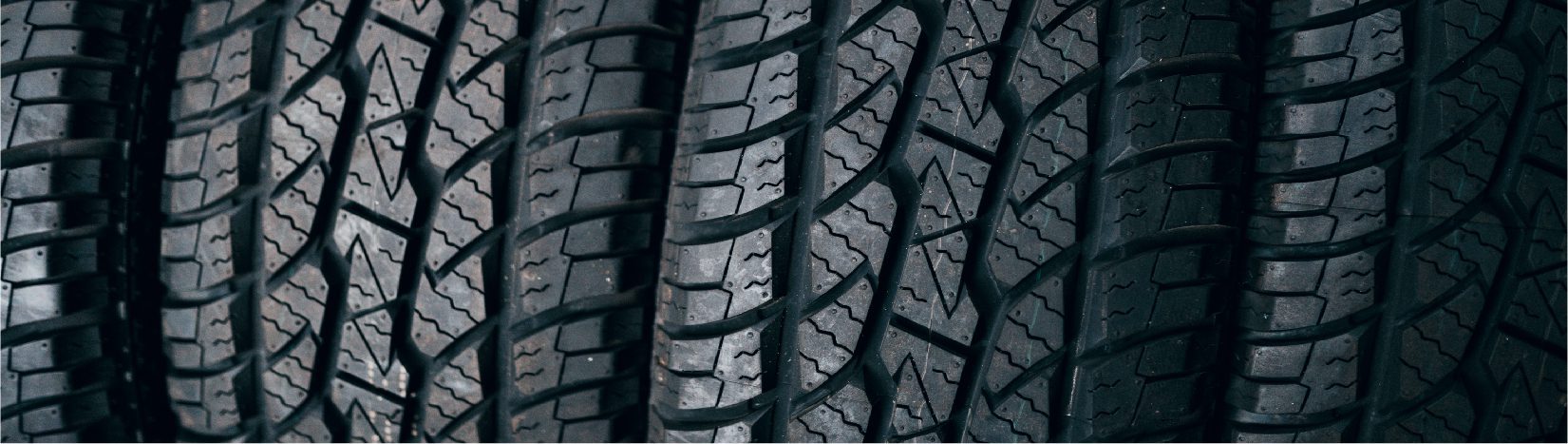
Prepared by
ANDREA HERRMANN
INTRODUCTION
Like all materials, rubber’s coefficient of friction is related in part to its surface roughness. In vehicle tire applications, traction with the road is very important. Surface roughness and the tire’s treads both play a role in this. In this study, the rubber surface and tread’s roughness and dimensions are analyzed.
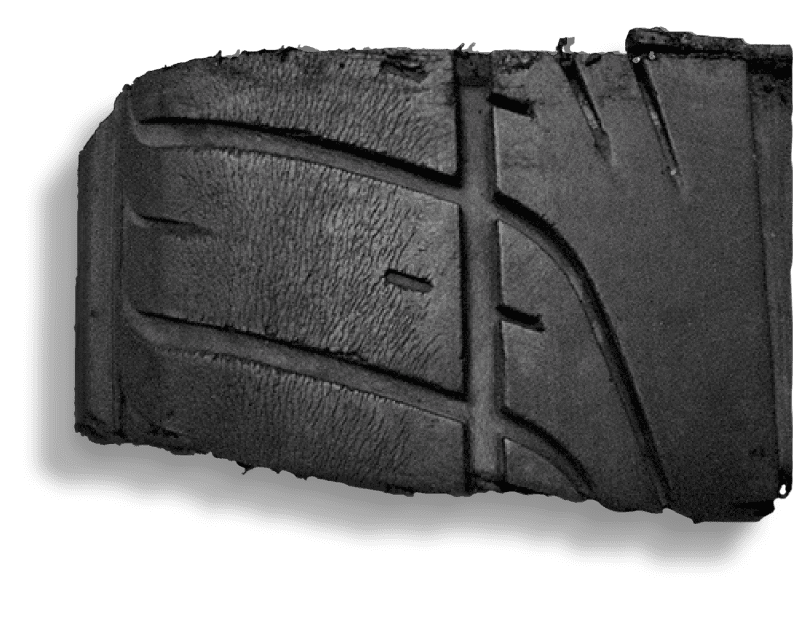
* THE SAMPLE
IMPORTANCE
OF 3D NON-CONTACT PROFILOMETRY
FOR RUBBER STUDIES
Unlike other techniques such as touch probes or interferometry, NANOVEA’s 3D Non-Contact Optical Profilers use axial chromatism to measure nearly any surface.
The Profiler system’s open staging allows for a wide variety of sample sizes and requires zero sample preparation. Nano through macro range features can be detected during a single scan with zero influence from sample reflectivity or absorption. Plus, these profilers have the advanced ability to measure high surface angles without requiring software manipulation of results.
Easily measure any material: transparent, opaque, specular, diffusive, polished, rough etc. The measurement technique of the NANOVEA 3D Non-Contact Profilers provides an ideal, broad and user friendly capability to maximize surface studies along with the benefits of combined 2D & 3D capability.
MEASUREMENT OBJECTIVE
In this application, we showcase the NANOVEA ST400, a 3D Non-Contact Optical Profiler measuring the surface and treads of a rubber tire.
A sample surface area large enough to represent the entire tire surface was selected at random for this study.
To quantify the rubber’s characteristics, we used the NANOVEA Ultra 3D analysis software to measure the contour dimensions, depth, roughness and developed area of the surface.
NANOVEA
ST400
The 3D View and False Color View of the treads show the value of mapping 3D surface designs. It provides users a straightforward tool to directly observe the size and shape of the treads from different angles. The Advanced Contour Analysis and Step Height Analysis are both extremely powerful tools for measuring precise dimensions of sample shapes and design
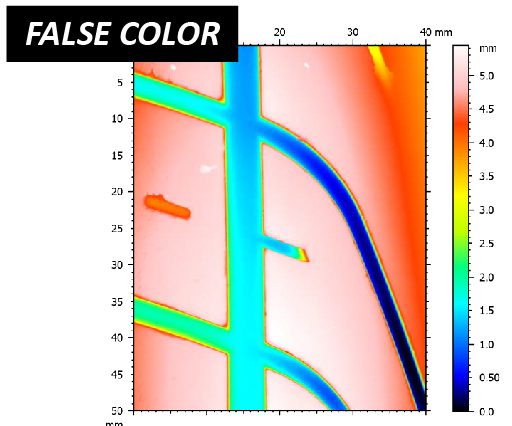
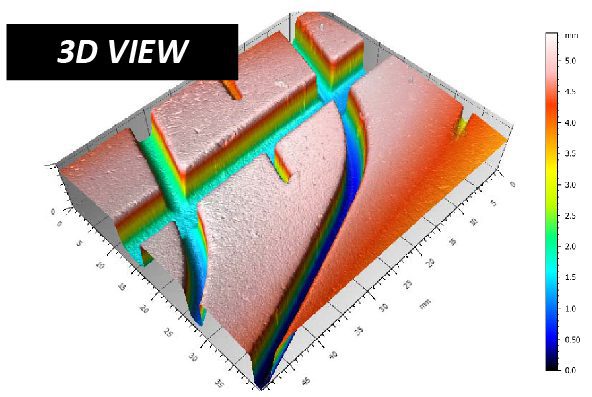
ADVANCED CONTOUR ANALYSIS
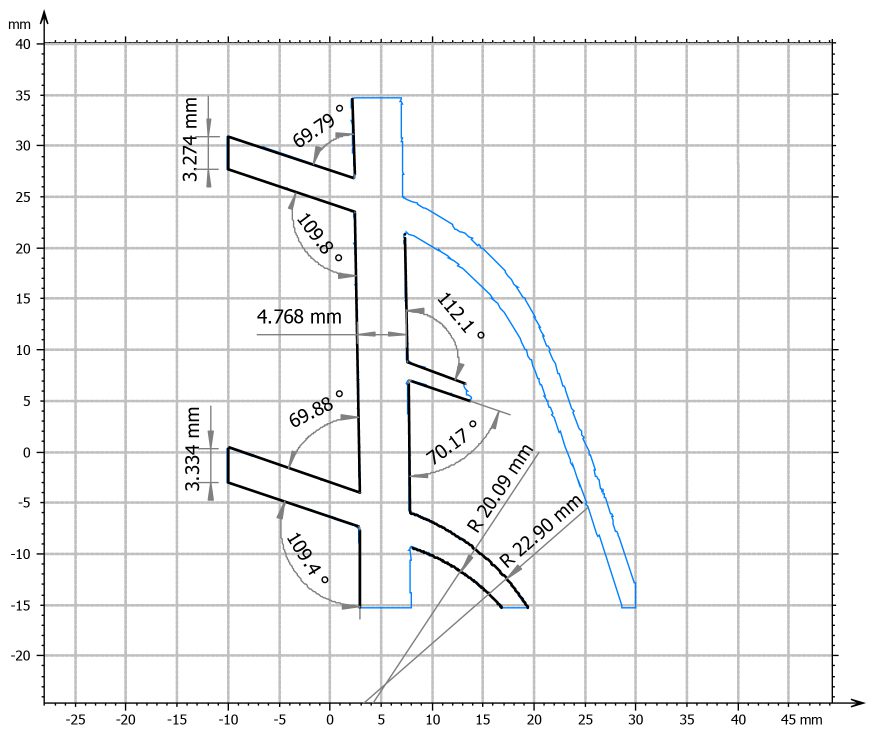
STEP HEIGHT ANALYSIS

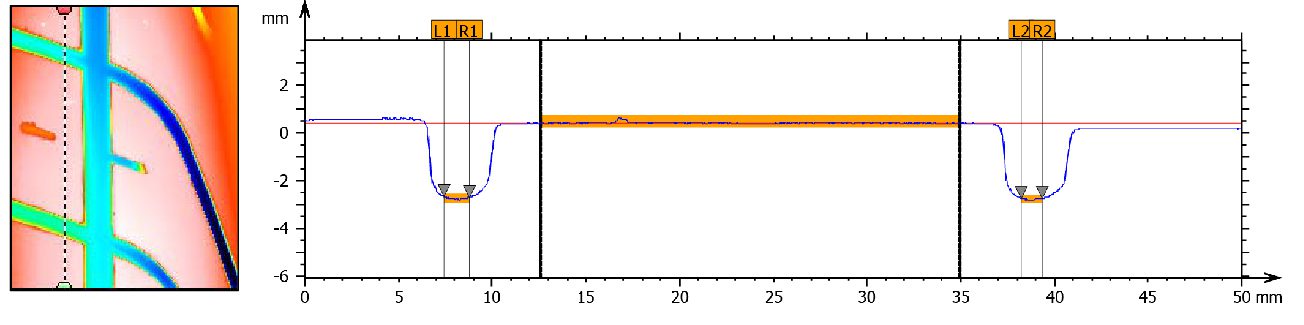
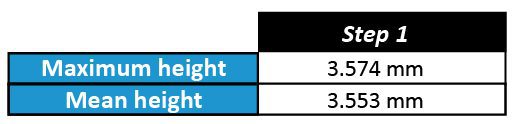
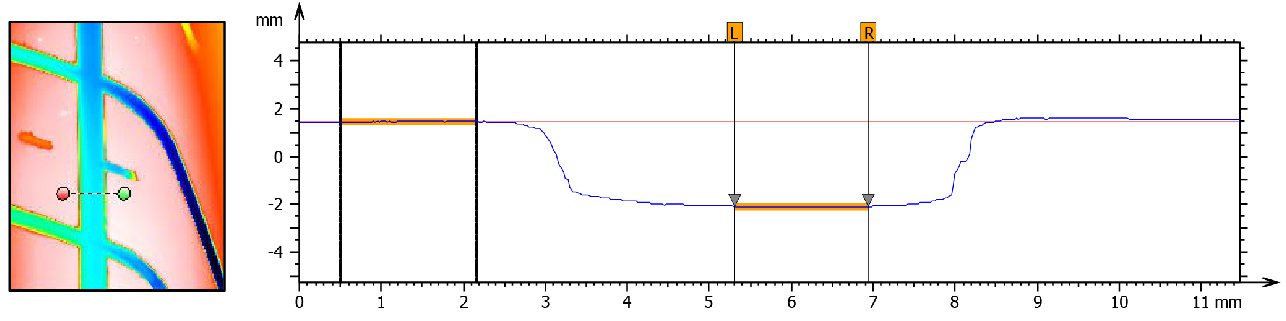
The rubber surface can be quantified in numerous ways using built-in software tools as shown in the following figures as examples. It can be observed that the surface roughness is 2.688 μm, and the developed area vs. projected area is 9.410 mm² vs. 8.997 mm². This information allows us to examine the relationship between surface finish and the traction of different rubber formulations or even rubber with varying degrees of surface wear.
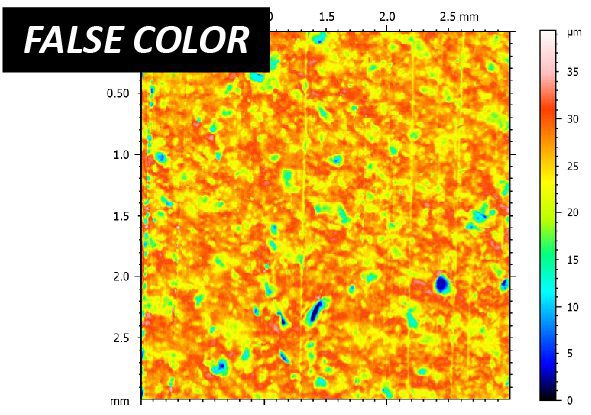
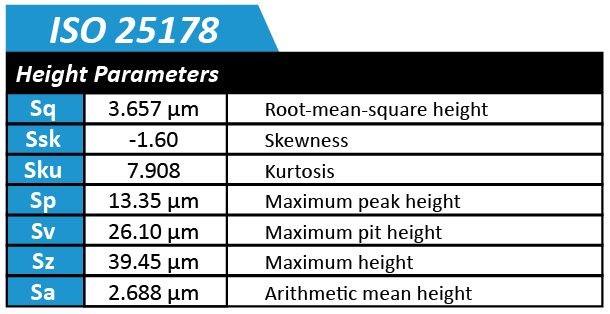
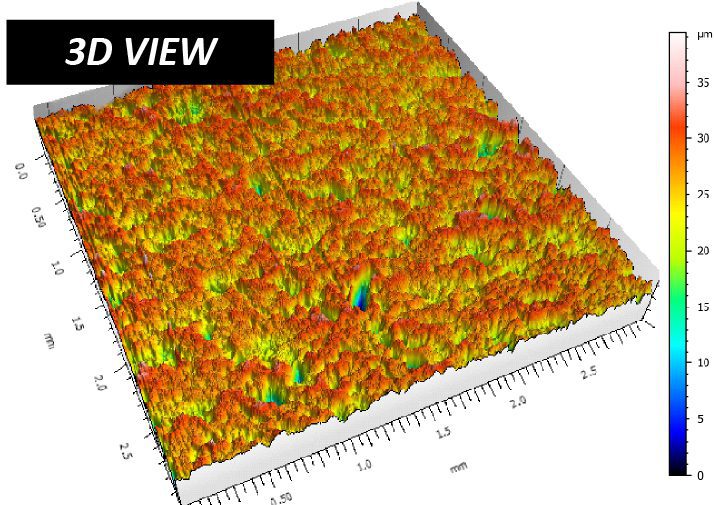
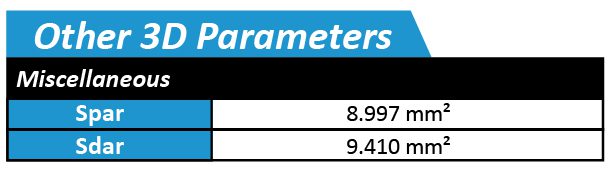
CONCLUSION
In this application, we have shown how the NANOVEA 3D Non-Contact Optical Profiler can precisely characterize the surface roughness and tread dimensions of rubber.
The data shows a surface roughness of 2.69 µm and a developed area of 9.41 mm² with a projected area of 9 mm². Various dimensions and radii of the rubber treads were measured as well.
The information presented in this study can be used to compare the performance of rubber tires with different tread designs, formulations, or varying degrees of wear. The data shown here represents only a portion of the calculations available in the Ultra 3D analysis software.
NOW, LET'S TALK ABOUT YOUR APPLICATION

Fresnel Lens Topography
FRESNEL LENS
DIMENSIONS USING 3D PROFILOMETRY
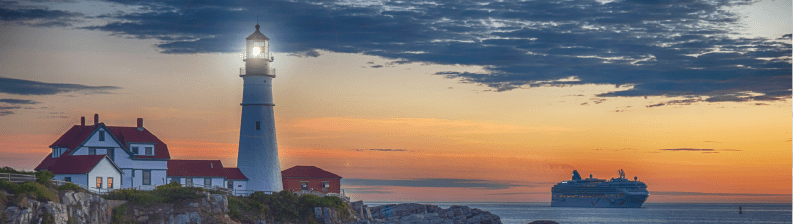
Prepared by
Duanjie Li & Benjamin Mell
INTRODUCTION
A lens is an optical device of axial symmetry that transmits and refracts light. A simple lens consists of a single optical component for converging or diverging the light. Even though spherical surfaces are not ideal shape for making a lens, they are often used as the simplest shape which glass can be ground and polished to.
A Fresnel lens consists of a series of concentric rings, which are thin parts of a simple lens with a width as small as a few thousandths of an inch. Fresnel lenses contain a large aperture and short focal length, with a compact design reducing the weight and volume of material required, compared to conventional lenses with the same optical properties. A very small amount of light is lost by absorption due to the thin geometry of the Fresnel lens.
IMPORTANCE OF 3D NON-CONTACT PROFILOMETRY FOR FRESNEL LENS INSPECTION
Fresnel lenses are extensively employed in the automotive industry, lighthouses, solar energy and optical landing systems for aircraft carriers. Molding or stamping the lenses out of transparent plastics can make their production cost-effective. Service quality of Fresnel lenses mostly depends on the precision and surface quality of their concentric ring. Unlike a touch probe technique, NANOVEA Optical Profilers perform 3D surface measurements without touching the surface, avoiding the risk of making new scratches. The Chromatic Light technique is ideal for precise scanning of complex shapes, such as lenses of different geometries.
FRESNEL LENS SCHEMATIC
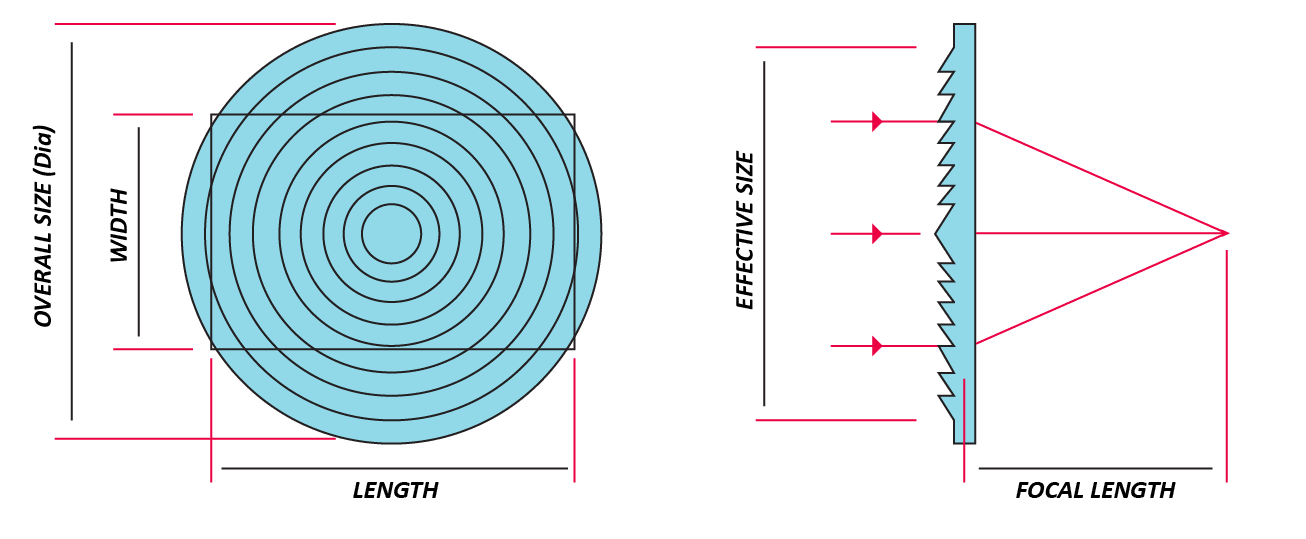
Transparent plastic Fresnel lenses can be manufactured by molding or stamping. Accurate and efficient quality control is critical to reveal defective production molds or stamps. By measuring the height and pitch of the concentric rings, production variations can be detected by comparing the measured values against the specification values given by the manufacturer of the lens.
Precise measurement of the lens profile ensures that the molds or stamps are properly machined to fit manufacturer specifications. Moreover, the stamp could progressively wear out over time, causing it to lose its initial shape. Consistent deviation from the lens manufacturer specification is a positive indication that the mold needs to be replaced.
MEASUREMENT OBJECTIVE
NANOVEA
ST400
The 2.3” x 2.3” acrylic Fresnel lens used for this study consists of
a series of concentric rings and a complex serrated cross-section profile.
It has a 1.5” focal length, 2.0” effective size diameter,
125 grooves per inch, and an index of refraction of 1.49.
The NANOVEA ST400 scan of the Fresnel lens shows a noticeable increase in height of the concentric rings, moving outward from the center.
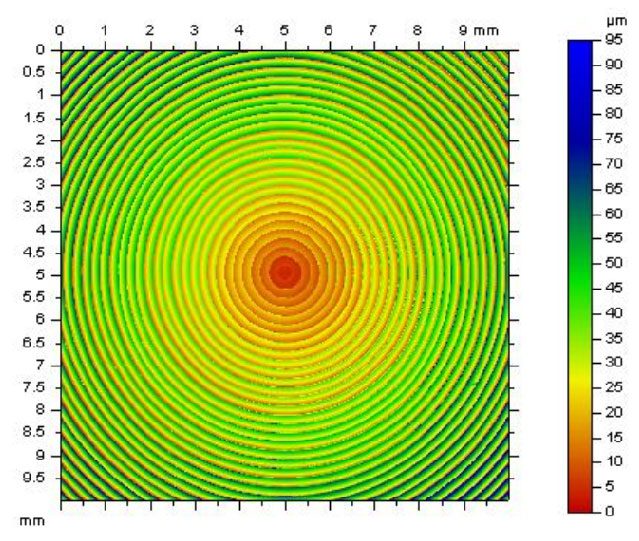
2D FALSE COLOR
Height Representation
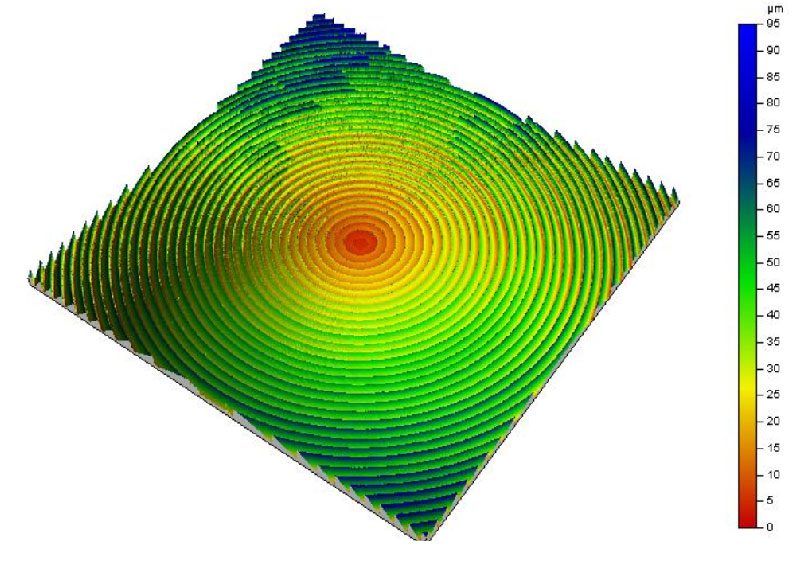
3D VIEW
EXTRACTED PROFILE
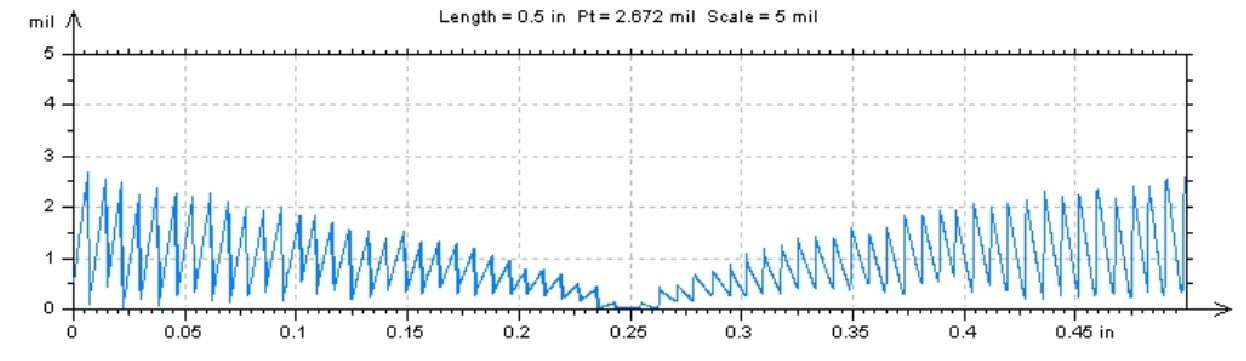
PEAK & VALLEY
Dimensional Analysis of the Profile
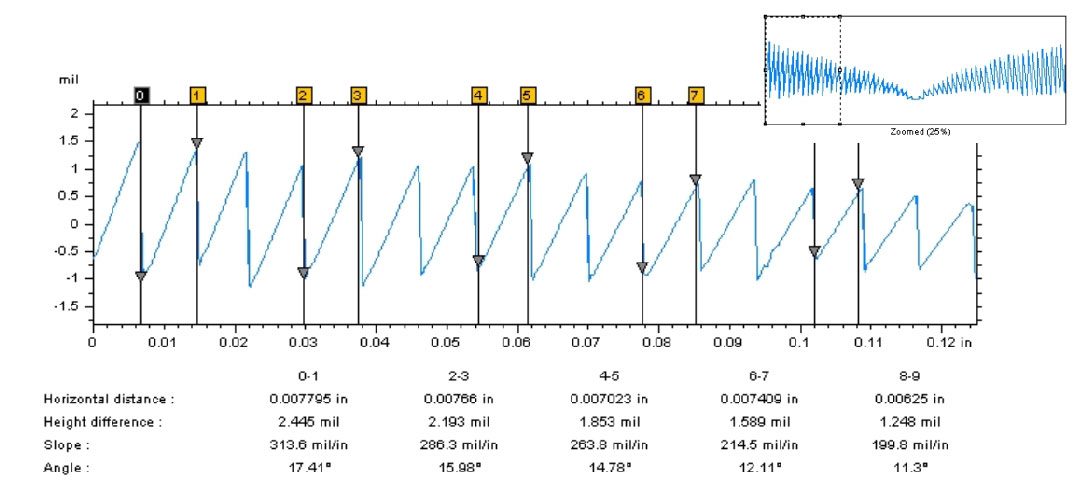
CONCLUSION
In this application, we have showcased that the NANOVEA ST400 non-contact Optical Profiler accurately measures the surface topography of Fresnel lenses.
The dimension of the height and pitch can be accurately determined from the complex serrated profile using NANOVEA analysis software. Users can effectively inspect the quality of the production molds or stamps by comparing the ring height and pitch dimensions of manufactured lenses against the ideal ring specification.
The data shown here represents only a portion of the calculations available in the analysis software.
NANOVEA Optical Profilers measure virtually any surface in fields including Semiconductors, Microelectronics, Solar, Fiber Optics, Automotive, Aerospace, Metallurgy, Machining, Coatings, Pharmaceutical, Biomedical, Environmental and many others.
NOW, LET'S TALK ABOUT YOUR APPLICATION

Pharmaceutical Tablets Surface Roughness Inspection
Introduction
Pharmaceutical tablets are the most popular medicinal dosage used today. Each tablet is made up by a combination of active substances (the chemicals that produce pharmacological effect) and inactive substances (disintegrant, binder, lubricant, diluent – usually in the form of powder). The active and inactive substances are then compressed or molded into a solid. Then, depending on the manufacturer specifications, the tablets are either coated or uncoated.
To be effective, tablet coatings need to follow the fine contours of embossed logos or characters on tablets, they need to be stable and sturdy enough to survive handling of the tablet, and they must not cause the tablets to stick to each other during the coating process. Current tablets typically have a polysaccharide and polymer-based coating which include substances like pigments and plasticizers. The two most common types of table coatings are film coatings and sugar coating. Compared to sugar coatings, film coatings are less bulky, more durable, and are less time-consuming to prepare and apply. However, film coatings have more difficulty hiding tablet appearance.
Tablet coatings are essential for moisture protection, masking the taste of the ingredients, and making the tablets easier to swallow. More importantly, the tablet coating controls the location and the rate in which the drug is released.
MEASUREMENT OBJECTIVE
In this application, we use the NANOVEA Optical Profiler and advanced Mountains software to measure and quantify the topography of various name brand pressed pills (1 coated and 2 uncoated) to compare their surface roughness.
It is assumed that Advil (coated) will have the lowest surface roughness due to the protective coating it has.
NANOVEA
HS2000
Test Conditions
Three batches of name brand pharmaceutical pressed tablets were scanned with the Nanovea HS2000
using High-Speed Line Sensor to measure various surface roughness parameters according to ISO 25178.
Scan Area
2 x 2 mm
Lateral Scan Resolution
5 x 5 μm
Scan Time
4 sec
Samples

Results & Discussion
After scanning the tablets, a surface roughness study was conducted with the advanced Mountains analysis software to calculate the surface average, root-mean-square, and maximum height of each tablet.

The calculated values support the assumption that Advil has a lower surface roughness due to the protective coating encasing its ingredients. Tylenol shows to have the highest surface roughness out of all three measured tablets.
A 2D and 3D height map of each tablet’s surface topography was produced which show the height distributions measured. One out of the five tablets were selected to represent the height maps for each brand. These height maps make a great tool for visual detection of outlying surface features such as pits or peaks.

Conclusion
In this study, we analyzed and compared the surface roughness of three name brand pressed pharmaceutical pills: Advil, Tylenol, and Excedrin. Advil proved to have the lowest average surface roughness. This can be attributed to the presence of the orange coating incasing the drug. In contrast, both Excedrin and Tylenol lack coatings, however, their surface roughness still differ from each other. Tylenol proved to have the highest average surface roughness out of all the tablets studied.
Using the NANOVEA HS2000 with High-Speed Line Sensor, we were able to measure 5 tablets in less than 1 minute. This can prove to be useful for quality control testing of hundreds of pills in a production today.
NOW, LET'S TALK ABOUT YOUR APPLICATION
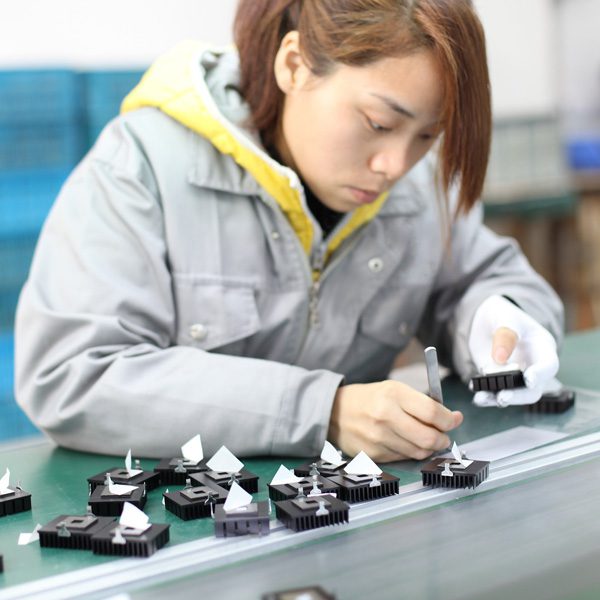
In-line Roughness Inspection
Learn more
IMPORTANCE OF NON-CONTACT PROFILER FOR IN-LINE ROUGHNESS INSPECTION
Surface defects derive from materials processing and product manufacturing. In-line surface quality inspection ensures the tightest quality control of the end products. The Nanovea 3D Non-Contact Profilometers utilize chromatic confocal technology with a unique capability to determine the roughness of a sample with-out contact. Multiple profiler sensors can be installed to monitor the roughness and texture of different areas of the product at the same time. The roughness threshold calculated in real-time by the analysis software serves as a fast and reliable pass/fail tool.
MEASUREMENT OBJECTIVE
In this study, the Nanovea roughness inspection conveyor system equipped with a point sensor is used to inspect the surface roughness of the acrylic and sandpaper samples. We showcase the capacity of Nanovea non-contact profilometer in providing fast and reliable in-line roughness inspection in a production line in real-time.
RESULTS AND DISCUSSION
The conveyor profilometer system can operate in two modes, namely Trigger Mode and Continuous Mode. As illustrated in Figure 2, the surface roughness of the samples are measured when they are passing under the optical profiler heads under the Trigger Mode. In comparison, Continuous Mode provides non-stop measurement of the surface roughness on the continuous sample, such as metal sheet and fabric. Multiple optical profiler sensors can be installed to monitor and record the roughness of different sample areas.
During the real-time roughness inspection measurement, the pass and fail alerts are displayed on the software windows as shown in Figure 4 and Figure 5. When the roughness value is within the given thresholds, the measured roughness is highlighted in green color. However, the highlight turns red when the measured surface roughness is out of the range of the set threshold values. This provides a tool for the user to determine the quality of a product’s surface finish.
In the following sections, two types of samples, e.g. Acrylic and Sandpaper are used to demonstrate the Trigger and Continuous Modes of the Inspection system.
Trigger Mode: Surface inspection of the Acrylic Sample
A series of Acrylic samples are aligned on the conveyor belt and move under the optical profiler head as shown in Figure 1. The false color view in Figure 6 shows the change of the surface height. Some of the mirror-like finished Acrylic samples had been sanded to create a rough surface texture as shown in Figure 6b.
As the Acrylic samples move at a constant speed under the optical profiler head, the surface profile is measured as shown in Figure 7 and Figure 8. The roughness value of the measured profile is calculated at the same time and compared to the threshold values. The red fail alert is launched when the roughness value is over the set threshold, allowing users to immediately detect and locate the defective product on the production line.
Continuous Mode: Surface Inspection of the sandpaper sample
Surface Height Map, Roughness Distribution Map, and Pass / Fail Roughness Threshold Map of the sandpaper sample surface as shown in Figure 9. The sandpaper sample has a couple of higher peaks in the used part as shown in the surface height map. The different colors in the pallet of Figure 9C represent the roughness value of the local surface. The Roughness Map exhibits a homogeneous roughness in the intact area of the sandpaper sample, while the used area is highlighted in dark blue color, indicating the reduced roughness value in this region. A Pass/Fail roughness threshold can be set up to locate such regions as shown in Figure 9D.
As the sandpaper continuously passes under the in-line profiler sensor, the real-time local roughness value is calculated and recorded as plotted in Figure 10. The pass/fail alerts are displayed on the software screen based on the set roughness threshold values, serving as a fast and reliable tool for quality control. The product surface quality in the production line is inspected in situ to discover defective areas in time.
In this application, we have shown the Nanovea Conveyor Profilometer equipped with an optical non-contact profiler sensor works as a reliable in-line quality control tool effectively and efficiently.
The inspection system can be installed in the production line to monitor the surface quality of the products in situ. The roughness threshold works as a dependable criteria to determine the surface quality of the products, allowing users to notice the defective products in time. Two inspection modes, namely Trigger Mode and Continuous Mode, are provided to meet the requirement for inspection on different types of products.
The data shown here represent only a portion of the calculations available in the analysis software. Nanovea Profilometers measure virtually any surface in fields including Semiconductor, Microelectronics, Solar, Fiber, Optics, Automotive, Aerospace, Metallurgy, Machining, Coatings, Pharmaceutical, Biomedical, Environmental and many others.
NOW, LET'S TALK ABOUT YOUR APPLICATION
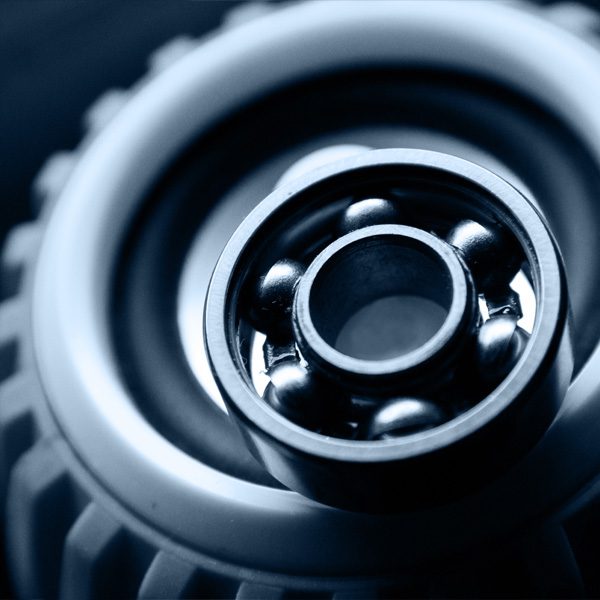
Block-On-Ring Wear Test
IMPORTANCE OF BLOCK-ON-RING WEAR EVALUATION
Block-on-Ring (ASTM G77) test is a widely used technique that evaluates the sliding wear behaviors of materials in different simulated conditions, allows reliable ranking of material couples for specific tribological applications.
MEASUREMENT OBJECTIVE
In this application, the Nanovea Mechanical Tester measures the YS and UTS of stainless steel SS304 and aluminum Al6061 metal alloy samples. The samples were chosen for their commonly recognized YS and UTS values showing the reliability of Nanovea’s indentation methods.
The sliding wear behavior of an H-30 block on an S-10 ring was evaluated by Nanovea’s tribometer using the Block-on-Ring module. The H-30 block is made of 01 tool steel of 30HRC hardness, while the S-10 ring is steel type 4620 of surface hardness 58 to 63 HRC and ring diameter of ~34.98 mm. Block-on-Ring tests were performed in dry and lubricated environments to investigate the effect on wear behavior. Lubrication tests were performed in USP heavy mineral oil. The wear track was examined using Nanovea’s 3D non-contact profilometer. Test parameters are summarized in Table 1. The wear rate (K), was evaluated using the formula K=V/(F×s), where V is the worn volume, F is the normal load, s is the sliding distance.
RESULTS AND DISCUSSION
Figure 2 compares the coefficient of friction (COF) of the Block-on-Ring tests in dry and lubricated environments. The block has significantly more friction in a dry environment than a lubricated environment. COF
fluctuates during the run-in period in the first 50-revolution and reaches a constant COF of ~0.8 for the rest of the 200-revolution wear test. In comparison, the Block-on-Ring test performed in the USP heavy mineral oil lubrication exhibits constant low COF of 0.09 throughout the 500,000-revolution wear test. The lubricant significantly reduces the COF between the surfaces by ~90 times.
Figures 3 and 4 show the optical images and cross-section 2D profiles of the wear scars on the blocks after dry and lubricated wear tests. The wear track volumes and wear rates are listed in Table 2. The steel block after the dry wear test at a lower rotational speed of 72 rpm for 200 revolutions exhibits a large wear scar volume of 9.45 mm˙. In comparison, the wear test carried out at a higher speed of 197 rpm for 500,000 revolutions in the mineral oil lubricant creates a substantially smaller wear track volume of 0.03 mm˙.
The images in ÿgure 3 show severe wear takes place during tests in the dry conditions compared to the mild wear from the lubricated wear test. High heat and intense vibrations generated during the dry wear test promotes oxidation of metallic debris resulting in severe three-body abrasion. In the lubricated test the mineral oil reduces friction and cools the contact face as well as transporting abrasive debris created during wear away. This leads to signiÿcant reduction of wear rate by a factor of ~8×10ˆ. Such a substantial di˛erence in wear resistance in di˛erent environments shows the importance of proper sliding wear simulation in realistic service conditions.
Wear behavior can change drastically when small changes in test conditions are introduced. The versatility of Nanovea’s tribometer allows wear measurement in high temperature, lubrication, and tribocorrosion conditions. The accurate speed and position control by the advanced motor enables wear tests to be performed at speeds ranging from 0.001 to 5000 rpm, making it an ideal tool for research/testing labs to investigate the wear in di˛erent tribological conditions.
The surface condition of the samples was examined by Nanovea’s non-contact optical proÿlometer. Figure 5 shows the surface morphology of the rings after the wear tests. The cylinder form is removed to better present the surface morphology and roughness created by the sliding wear process. Signiÿcant surface roughening took place due to the three-body abrasion process during the dry wear test of 200 revolutions. The block and ring after the dry wear test exhibit a roughness Ra of 14.1 and 18.1 µm, respectively, compared to 5.7 and 9.1 µm for the long-term 500,000 – revolution lubricated wear test at a higher speed. This test demonstrates the importance of proper lubrication of piston ring-cylinder contact. Severe wear quickly damages the contact surface without lubrication and leads to irreversible deterioration of the service quality and even breakage of the engine.
CONCLUSION
In this study we showcase how Nanovea’s Tribometer is used to evaluate the sliding wear behavior of a steel metal couple using the Block-on-Ring module following the ASTM G77 Standard. The lubricant plays a critical role in the wear properties of the material couple. The mineral oil reduces the wear rate of the H-30 block by a factor of ~8×10ˆ and the COF by ~90 times. The versatility of Nanovea’s Tribometer makes it an ideal tool for measuring wear behavior under various lubrication, high temperature, and tribocorrosion conditions.
Nanovea’s Tribometer o˛ers precise and repeatable wear and friction testing using ISO and ASTM compliant rotative and linear modes, with optional high-temperature wear, lubrication, and tribo-corrosion modules available in one pre-integrated system. Nanovea’s unmatched range is an ideal solution for determining the full range of tribological properties of thin or thick, soft, or hard coatings, ÿlms, and substrates.
NOW, LET'S TALK ABOUT YOUR APPLICATION
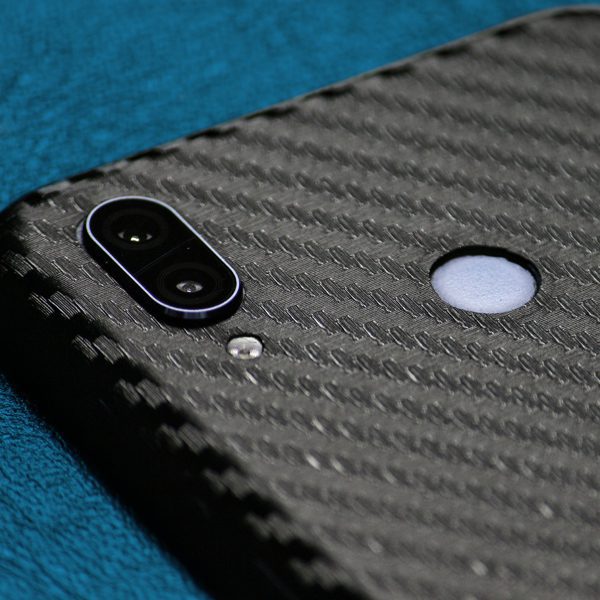
Composite Material Analysis using 3D Profilometry
Importance of Non-Contact Profilometry for Composite Materials
It is crucial defects are minimized so composite materials are as strong as possible in reinforcement applications. As an anisotropic material, it is critical weave direction is consistent to maintain high performance predictability. Composite materials have one of the highest strength to weight ratios making it stronger than steel in some cases. It is important to limit exposed surface area in composites to minimize chemical vulnerability and thermal expansion effects. Profilometry surface inspection is critical to quality control production of composites to ensure strong performance over a long service time.
Nanovea’s 3D Non-Contact Profilometer is unlike other surface measurement techniques such as touch probes or interferometry. Our profilometers use axial chromatism to measure nearly any surface and open staging allows for samples of any size with no preparation needed. Nano through macro measurements are obtained during surface profile measurement with zero influence from sample reflectivity or absorption. Our profilometers easily measure any material: transparent, opaque, specular, diffusive, polished, and rough with the advanced ability to measure high surface angles with no software manipulation. The Non-Contact Profilometer technique provides the ideal and user-friendly capability to maximize composite material surface studies; along with the benefits of combined 2D & 3D capability.
Measurement Objective
The Nanovea HS2000L Profilometer used in this application measured the surface of two weaves of carbon fiber composites. Surface roughness, weave length, isotropy, fractal analysis, and other surface parameters are used to characterize the composites. The area measured was randomly selected and assumed large enough that property values can be compared using Nanovea’s powerful surface analysis software.
Results and Discussion
Surface Analysis
Isotropy shows directionality of the weave to determine expected property values. Our study shows how the bidirectional composite is ~60% isotropic as expected. Meanwhile, the unidirectional composite is ~13% isotropic due to the strong single fiber path direction fiber.
Weave size determines the consistency of packing and width of fibers used in the composite. Our study shows how easily we can measure weave size down to micron accuracy to ensure quality parts.


Texture analysis of the dominant wavelength suggests strand size for both composites are 4.27 microns thick. Fractal dimension analysis of the fiber surface determines smoothness to find how easily fibers will set in a matrix. The fractal dimension of the unidirectional fiber is higher than the bidirectional fiber which may affect the processing of composites.
Conclusion
In this application, we have shown the Nanovea HS2000L Non-Contact Profilometer precisely characterizes the fibrous surface of composite materials. We distinguished differences between weave types of carbon fiber with height parameters, isotropy, texture analysis, and distance measurements along with much more.
Our profilometer surface measurements precisely and quickly mitigate composite damage which decreases defects in parts, maximizing composite material capability. Nanovea’s 3D profilometer speed ranges from <1mm/s to 500mm/s for suitability in research applications to the needs of high-speed inspection. The Nanovea profilometer is the solution
to any composite measurement need.
NOW, LET'S TALK ABOUT YOUR APPLICATION
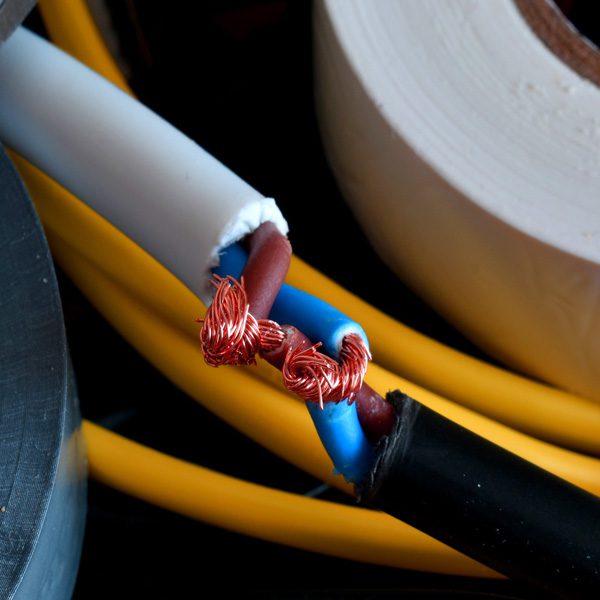
Wear and Scratch Evaluation of Surface Treated Copper Wire
Importance of Wear and Scratch Evaluation of Copper Wire
Copper has a long history of use in electric wiring since the invention of the electromagnet and telegraph. Copper wires are applied in a wide range of electronic equipment such as panels, meters, computers, business machines, and appliances thanks to its corrosion resistance, solderability, and performance at elevated temperatures up to 150°C. Approximately half of all mined copper is used for manufacturing electrical wire and cable conductors.
Copper wire surface quality is critical to application service performance and lifetime. Micro defects in wires may lead to excessive wear, crack initiation and propagation, decreased conductivity, and inadequate solderability. Proper surface treatment of copper wires removes surface defects generated during wire drawing improving corrosion, scratch, and wear resistance. Many aerospace applications with copper wires require controlled behavior to prevent unexpected equipment failure. Quantifiable and reliable measurements are needed to properly evaluate the wear and scratch resistance of the copper wire surface.
Measurement Objective
In this application we simulate a controlled wear process of different copper wire surface treatments. Scratch testing measures the load required to cause failure on the treated surface layer. This study showcases the Nanovea Tribometer and Mechanical Tester as ideal tools for evaluation and quality control of electric wires.
Test Procedure and Procedures
Coefficient of friction (COF) and wear resistance of two different surface treatments on copper wires (Wire A and Wire B) were evaluated by the Nanovea tribometer using a linear reciprocating wear module. An Al₂O₃ ball (6 mm diameter) is the counter material used in this application. The wear track was examined using Nanovea’s 3D non-contact profilometer. Test parameters are summarized in Table 1.
A smooth Al₂O₃ ball as a counter material was used as an example in this study. Any solid material with different shape and surface finish can be applied using a custom fixture to simulate the actual application situation.
Results and Discussion
Wear of copper wire:
Figure 2 shows COF evolution of the copper wires during wear tests. Wire A shows a stable COF of ~0.4 throughout the wear test while wire B exhibits a COF of ~0.35 in the first 100 revolutions and progressively increases to ~0.4.
Figure 3 compares wear tracks of the copper wires after tests. Nanovea’s 3D non-contact profilometer offered superior analysis of the detailed morphology of wear tracks. It allows direct and accurate determination of the wear track volume by providing a fundamental understanding of the wear mechanism. Wire B’s surface has signi¬ficant wear track damage after a 600-revolution wear test. The profilometer 3D view shows the surface treated layer of Wire B removed completely which substantially accelerated the wear process. This left a flattened wear track on Wire B where copper substrate is exposed. This may result in significantly shortened lifespan of electrical equipment where Wire B is used. In comparison, Wire A exhibits relatively mild wear shown by a shallow wear track on the surface. The surface treated layer on Wire A did not remove like the layer on Wire B under the same conditions.
Scratch resistance of the copper wire surface:
Figure 4 shows the scratch tracks on the wires after testing. The protective layer of Wire A exhibits very good scratch resistance. It delaminates at a load of ~12.6 N. In comparison, the protective layer of Wire B failed at a load of ~1.0 N. Such a significant difference in scratch resistance for these wires contributes to their wear performance, where Wire A possesses substantially enhanced wear resistance. The evolution of normal force, COF, and depth during the scratch tests shown in Fig. 5 provides more insight on coating failure during tests.
Conclusion
In this controlled study we showcased the Nanovea’s tribometer conducting quantitative evaluation of wear resistance for surface treated copper wires and Nanovea’s mechanical tester providing reliable assessment of copper wire scratch resistance. Wire surface treatment plays a critical role in the tribo-mechanical properties during their lifetime. Proper surface treatment on Wire A significantly enhanced wear and scratch resistance, critical in the performance and lifespan of electrical wires in rough environments.
Nanovea’s tribometer offers precise and repeatable wear and friction testing using ISO and ASTM compliant rotative and linear modes, with optional high temperature wear, lubrication, and tribo-corrosion modules available in one pre-integrated system. Nanovea’s unmatched range is an ideal solution for determining the full range of tribological properties of thin or thick, soft or hard coatings, films, and substrates.
NOW, LET'S TALK ABOUT YOUR APPLICATION
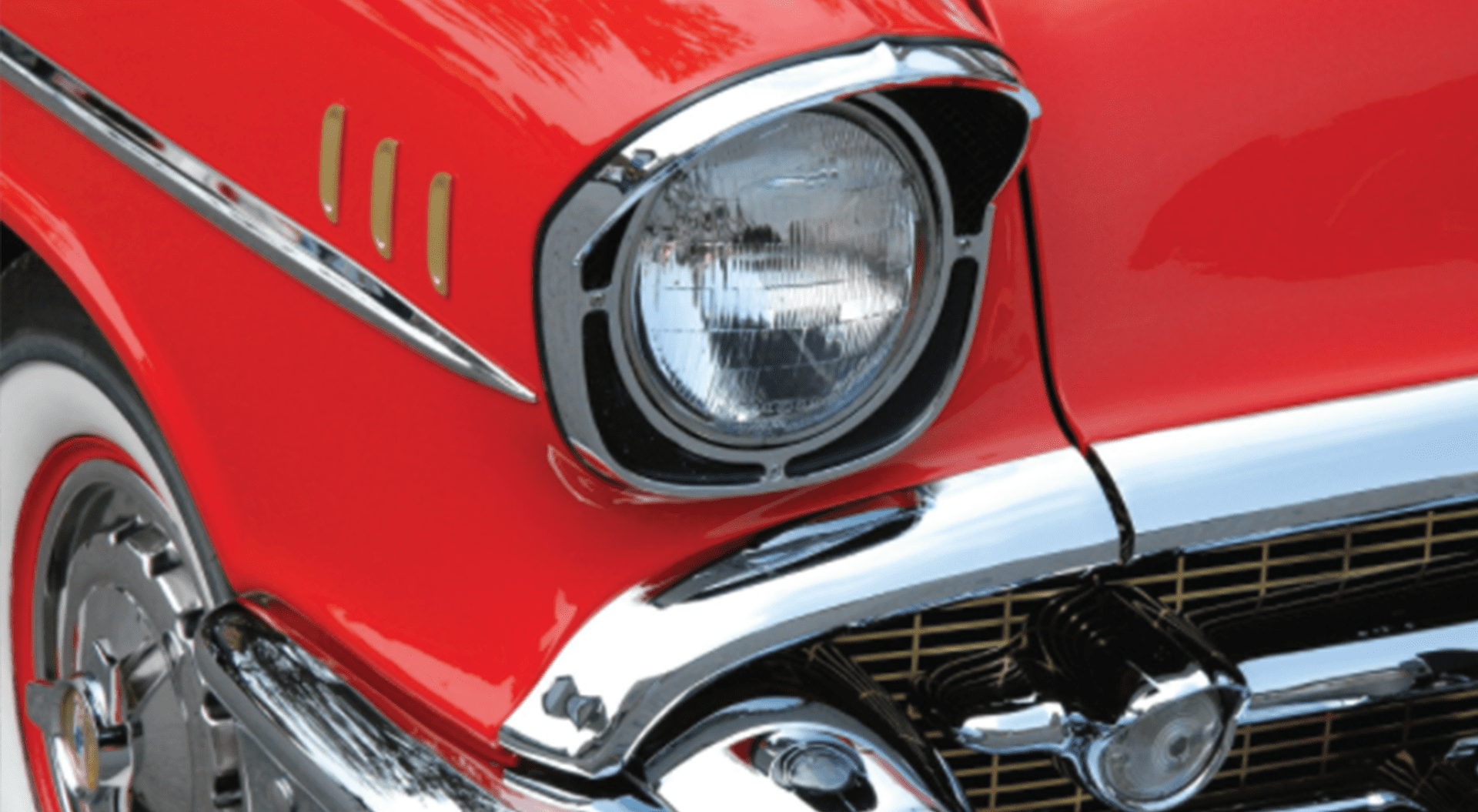
Paint Orange Peel Texture Analysis using 3D Profilometry
Paint Orange Peel Texture Analysis using 3D Profilometry
Introduction
The size and frequency of surface structures on substrates affect the quality of gloss coatings. Paint orange peel texture, named after its appearance, can develop from substrate influence and paint application technique. Texture problems are commonly quantified by waviness, wavelength, and the visual effect they have on gloss coatings. The smallest textures result in gloss reduction while larger textures result in visible ripples on the coated surface. Understanding the development of these textures and its relation to substrates and techniques are critical to quality control.
Importance of Profilometry for Texture Measurement
Unlike traditional 2D instruments used to measure gloss texture, 3D non-contact measurement quickly provides a 3D image used to understand surface characteristics with the added ability to quickly investigate areas of interest. Without speed and 3D review, a quality control environment would solely rely on 2D information that gives little predictability of the entire surface. Understanding textures in 3D allows for the best selection of processing and control measures. Assuring quality control of such parameters heavily relies on quantifiable, reproducible, and reliable inspection. Nanovea 3D Non-Contact Profilometers utilize chromatic confocal technology to have the unique capability to measure the steep angles found during fast measurement. Nanovea Profilometers succeed where other techniques fail to provide reliable data due to probe contact, surface variation, angle, or reflectivity.
Measurement Objective
In this application, the Nanovea HS2000L measures the paint orange peel texture of a gloss paint. There are endless surface parameters automatically calculated from the 3D surface scan. Here we analyze a scanned 3D surface by quantifying the characteristics of the paint orange peel texture.
The Nanovea HS2000L quantified isotropy and height parameters of the orange peel paint. The orange peel texture quantified the random pattern direction with 94.4% isotropy. Height parameters quantify the texture with a 24.84µm height difference.
The bearing ratio curve in Figure 4 is a graphical representation of the depth distribution. This is an interactive feature within the software that allows the user to view distributions and percentages at varying depths. An extracted profile in Figure 5 gives useful roughness values for the orange peel texture. Peak extraction above a 144 micron threshold shows the orange peel texture. These parameters are easily adjusted to other areas or parameters of interest.
Conclusion
In this application, the Nanovea HS2000L 3D Non-Contact Profilometer precisely characterizes both topography and nanometer details of the paint orange peel texture on a gloss coating. Areas of interest from 3D surface measurements are quickly identified and analyzed with many useful measurements (Dimension, Roughness Finish Texture, Shape Form Topography, Flatness Warpage Planarity, Volume Area, Step-Height, etc.). Quickly chosen 2D cross-sections provide a complete set of surface measurement resources on gloss texture. Special areas of interest can be further analyzed with an integrated AFM module. Nanovea 3D Profilometer’s speed ranges from <1 mm/s to 500 mm/s for suitability in research applications to the needs of high-speed inspection. Nanovea 3D Profilometers have a wide range of configurations to suit your application.
NOW, LET'S TALK ABOUT YOUR APPLICATION
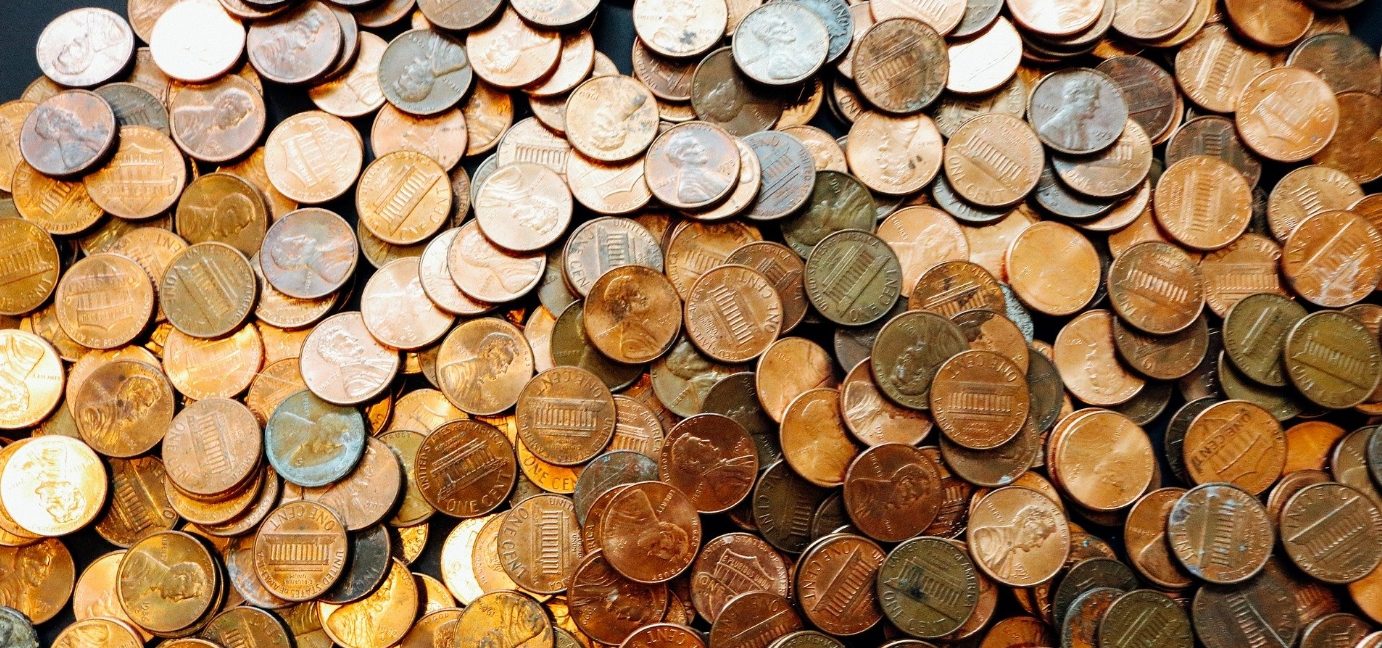
3D Surface Analysis of a Penny with Non-contact Profilometry
Importance of Non-contact Profilometry for Coins
Currency is highly valued in modern society because it is traded for goods and services. Coin and paper bill currency circulates around the hands of many people. Constant transfer of physical currency creates surface deformation. Nanovea’s 3D Profilometer scans the topography of coins minted in different years to investigate surface differences.
Coin features are easily recognizable to the general public since they are common objects. A penny is ideal for introducing the strength of Nanovea’s Advanced Surface Analysis Software: Mountains 3D. Surface data collected with our 3D Profilometer allows for high level analyses on complex geometry with surface subtraction and 2D contour extraction. Surface subtraction with a controlled mask, stamp, or mold compares the quality of manufacturing processes while contour extraction identifies tolerances with dimensional analysis. Nanovea’s 3D Profilometer and Mountains 3D software investigates the submicron topography of seemingly simple objects, like pennies.
Measurement Objective
The full upper surface of five pennies were scanned using Nanovea’s High-Speed Line Sensor. The inner and outer radius of each penny was measured using Mountains Advanced Analysis Software. An extraction from each penny surface at an area of interest with direct surface subtraction quantified surface deformation.
Results and Discussion
3D Surface
The Nanovea HS2000 profilometer took only 24 seconds to scan 4 million points in a 20mm x 20mm area with a 10um x 10um step size to acquire the surface of a penny. Below is a height map and 3D visualization of the scan. The 3D view shows the High-Speed sensor’s ability to pick up small details unperceivable to the eye. Many small scratches are visible across the surface of the penny. Texture and roughness of the coin seen in the 3D view are investigated.
The contours of the penny were extracted and dimensional analysis obtained inner and outer diameters of the edge feature. The outer radius averaged 9.500 mm ± 0.024 while the inner radius averaged 8.960 mm ± 0.032. Additional dimensional analyses Mountains 3D can do on 2D and 3D data sources are distance measurements, step height, planarity, and angle calculations.
Figure 5 shows the area of interest for the surface subtraction analysis. The 2007 penny was used as the reference surface for the four older pennies. Surface subtraction from the 2007 penny surface shows differences between pennies with holes/peaks. Total surface volume difference is obtained from adding volumes of the holes/peaks. The RMS error refers to how closely penny surfaces agree with each other.
Conclusion
Nanovea’s High-Speed HS2000L scanned five pennies minted in different years. Mountains 3D software compared surfaces of each coin using contour extraction, dimensional analysis, and surface subtraction. The analysis clearly defines the inner and outer radius between the pennies while directly comparing surface feature differences. With Nanovea’s 3D profilometer’s ability to measure any surfaces with nanometer-level resolution, combined with Mountains 3D analysis capabilities, the possible Research and Quality Control applications are endless.
NOW, LET'S TALK ABOUT YOUR APPLICATION
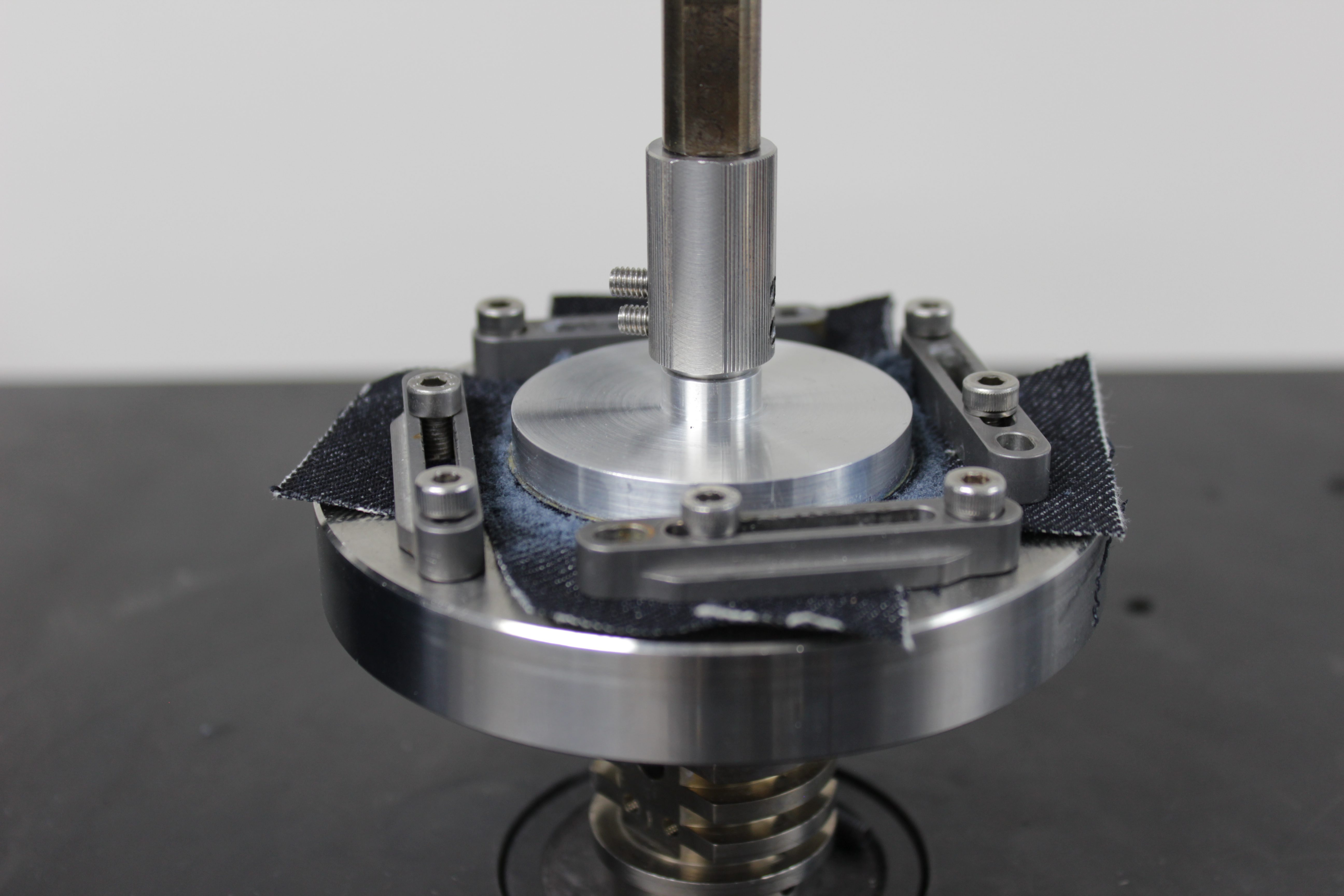
Comparing Abrasion Wear on Denim
Introduction
The form and function of a fabric is determined by its quality and durability. Daily usage of fabrics cause wear and tear on the material, e.g. piling, fuzzing, and discoloration. Subpar fabric quality used for clothing can often lead to consumer dissatisfaction and brand damage.
Attempting to quantify the mechanical properties of fabrics can pose many challenges. The yarn structure and even the factory in which it was produced can result in poor reproducibility of test results. Making it difficult to compare test results from different laboratories. Measuring the wear performance of fabrics is critical to the manufacturers, distributors, and retailers in the textile production chain. A well controlled and reproducible wear resistance measurement is crucial to ensure reliable quality control of the fabric.
Categories
- Application Notes
- Block on Ring Tribology
- Corrosion Tribology
- Friction Testing | Coefficient of Friction
- High Temperature Mechanical Testing
- High Temperature Tribology
- Humidity and Gases Tribology
- Humidity Mechanical Testing
- Indentation | Creep and Relaxation
- Indentation | Fracture Toughness
- Indentation | Hardness and Elastic
- Indentation | Loss and Storage
- Indentation | Stress vs Strain
- Indentation | Yield Strength and Fatigue
- Laboratory Testing
- Linear Tribology
- Liquid Mechanical Testing
- Liquid Tribology
- Low Temperature Tribology
- Mechanical Testing
- Press Release
- Profilometry | Flatness and Warpage
- Profilometry | Geometry and Shape
- Profilometry | Roughness and Finish
- Profilometry | Step Height and Thickness
- Profilometry | Texture and Grain
- Profilometry | Volume and Area
- Profilometry Testing
- Ring on Ring Tribology
- Rotational Tribology
- Scratch Testing | Adhesive Failure
- Scratch Testing | Cohesive Failure
- Scratch Testing | Multi-Pass Wear
- Scratch Testing | Scratch Hardness
- Scratch Testing Tribology
- Tradeshow
- Tribology Testing
- Uncategorized
Archives
- September 2023
- August 2023
- June 2023
- May 2023
- July 2022
- May 2022
- April 2022
- January 2022
- December 2021
- November 2021
- October 2021
- September 2021
- August 2021
- July 2021
- June 2021
- May 2021
- March 2021
- February 2021
- December 2020
- November 2020
- October 2020
- September 2020
- July 2020
- May 2020
- April 2020
- March 2020
- February 2020
- January 2020
- November 2019
- October 2019
- September 2019
- August 2019
- July 2019
- June 2019
- May 2019
- April 2019
- March 2019
- January 2019
- December 2018
- November 2018
- October 2018
- September 2018
- July 2018
- June 2018
- May 2018
- April 2018
- March 2018
- February 2018
- November 2017
- October 2017
- September 2017
- August 2017
- June 2017
- May 2017
- April 2017
- March 2017
- February 2017
- January 2017
- November 2016
- October 2016
- August 2016
- July 2016
- June 2016
- May 2016
- April 2016
- March 2016
- February 2016
- January 2016
- December 2015
- November 2015
- October 2015
- September 2015
- August 2015
- July 2015
- June 2015
- May 2015
- April 2015
- March 2015
- February 2015
- January 2015
- November 2014
- October 2014
- September 2014
- August 2014
- July 2014
- June 2014
- May 2014
- April 2014
- March 2014
- February 2014
- January 2014
- December 2013
- November 2013
- October 2013
- September 2013
- August 2013
- July 2013
- June 2013
- May 2013
- April 2013
- March 2013
- February 2013
- January 2013
- December 2012
- November 2012
- October 2012
- September 2012
- August 2012
- July 2012
- June 2012
- May 2012
- April 2012
- March 2012
- February 2012
- January 2012
- December 2011
- November 2011
- October 2011
- September 2011
- August 2011
- July 2011
- June 2011
- May 2011
- November 2010
- January 2010
- April 2009
- March 2009
- January 2009
- December 2008
- October 2008
- August 2007
- July 2006
- March 2006
- January 2005
- April 2004